A biomimetic sperm selection device for routine sperm selection
Steven A. Vasilescu , Dale M. Goss , Kathryn H. Gurner , Rebecca L. Kelley , Maria Mazi , Fabrice K. De Bond , Jennifer Lorimer , Fabrizzio Horta , Farin Y. Parast , David K. Gardner , Reza Nosrati , Majid E. Warkiani
Research question: Can a biomimetic microfluidic sperm-sorter isolate motile sperm while minimizing DNA damage, in comparison to density gradient centrifugation (DGC)?
Design: This is a two-phase study on 61 men, consisting of 21 donated semen samples in a university research laboratory, followed by a diagnostic andrology study on 40 consenting patients presenting at a fertility clinic for semen diagnostics. Each sample was split to perform DGC and microfluidic sperm selections (one step sperm selection with a 15 minute 2 incubation time) side-by-side. Outcomes evaluated included concentration, progressive motility, and DNA fragmentation index (DFI) between raw semen, DGC and microfluidic device. Results were analysed by Friedman’s test for non-parametric data (significant when P-value < 0.05). DFI was assessed by sperm chromatin dispersion assay (SCD).
Results: Both DGC and the microfluidic device showed improvements in sperm DFI and motility over the raw sample in both cohorts. However, the microfluidic device was significantly better than DGC at reducing DFI from the raw semen in both the proof-ofconcept (P < 0.01) and diagnostic andrology studies (P < 0.001). Progressive motility was significantly higher for the microfluidic device processing for the proof-of-concept study (P < 0.01) but not the diagnostic andrology study. Sperm concentration was significantly lower in the microfluidic device group versus the DGC group for both cohorts (P < 0.001).
Conclusions: Channel-based biomimetic sperm selection can passively select motile sperm with low DNA fragmentation. When compared to DGC, this method isolates fewer sperm with a higher proportion of progressively motile cells and with greater DNA integrity.
Keywords
Sperm Selection, Microfluidics, density gradient centrifugation, DNA Fragmentation
Key Message
A biomimetic microfluidic sperm-sorter proved more effective than conventional density gradient centrifugation (DGC) in isolating sperm with lower DNA fragmentation and higher motility in a two phase, 61-men study, offering a gentler and potentially more fertility-preserving method of sperm selection.
Introduction
Infertility affects approximately 15% of couples worldwide, with roughly 55% of those having a male contributing factor (WHO, 2023). The use of medical intervention in the form of assisted reproductive technology (ART) is growing yearly, yet the success rate of assisted reproduction cycles per embryo transfer has stagnated at ̴33% per cycle and the proportion of live birth is plateaued at ̴ 26% per cycle over the last two decades (Nosrati et al. 2017; Adamson et al. 2018). Many factors play a role in the success of a cycle, and one crucial aspect of all methods is sperm selection, where sperm quality can directly impact outcomes (Donnelly et al. 1998; Tandara et al. 2014).
Increased DNA fragmentation index (DFI) is prevalent in infertile men and in men over 40, and even higher in those with abnormalities in conventional semen parameters such as motility, morphology and concentration (Moskovtsev et al. 2009). Furthermore, couples with idiopathic infertility and recurrent pregnancy loss, where conventional sperm parameters lie within healthy norms, show a higher incidence of DNA fragmentation (Tan et al. 2019; Esteves et al. 2021). Studies have shown, using sperm chromatin structure assay (SCSA), that the number of men with moderate (>20%) or high (>30%) DFI is together approximately 28% of men from infertile couples (Erenpreiss et al. 2008), while in couples with ‘unexplained infertility’ the percentage of men with moderate to high DFI was 26.1% (Oleszczuk et al. 2013), and that this incidence increase with age. High levels of DNA fragmentation (>30%) in sperm have been shown to increase the risk factors involved in in vitro fertilization (IVF) by reducing embryo quality, lowering implantation rates, and increasing the chance of miscarriage up to 3.9 times that of patients using low DFI sperm (Duran et al. 2002; Robinson et al. 2012; Sedó et al. 2017; Borges Jr et al. 2019).
Density gradient centrifugation (DGC) and swim-up methods are the most commonly used techniques for processing semen samples and selecting sperm for use in assisted reproduction. However, recent studies, these methods have been implicated in the increase in sperm DNA fragmentation purportedly due to the generation of reactive oxygen species (ROS) (Stevanato et al. 2008; Aitken et al. 2014; Muratori et al. 2016). As it is currently not possible to assess sperm DNA fragmentation non-invasively prior to use in IVF or intracytoplasmic sperm injection (ICSI), innovative methods are required to select and isolate low DFI sperm for use in IVF or ICSI at appropriate concentrations. There have been several 4 attempts to create an alternative sperm selection method such as the MACS® ART Annexin V System (Miltenyi Biotec, Sydney, Australia), Physiological Intracytoplasmic Sperm Injection (PICSI® ) dish (CooperSurgical, Trumbull, USA) or the DGC-zeta potential method (Hasanen et al. 2020; Karimi et al. 2020), and many innovative approaches have originated from the application of microfluidics (Suh et al. 2006; Quinn et al. 2018; Keskin et al. 2021; Villeneuve et al. 2023).
Microfluidic devices have been developed to select high-quality sperm using flow or sperm migration behavior, without the need for active input such as centrifugation or electrophoretic fields. These devices purport to reduce exposure to oxidative stress and subsequent DNA fragmentation (Nosrati et al. 2014; Simchi et al. 2021; Dadkhah et al. 2023). However, the clinical translation and adoption of many of these technologies have been limited (Nosrati 2022), largely due to their complexity of workflow, operational instability, and/or inconsistent results. Without an intuitive user interface, many devices have not seen further side-by-side clinical testing to evaluate their performance in the hands of clinical scientists. An effective sperm selection platform must not only provide high-quality sperm in a timely manner but must also be simple to use and consistent in its performance over a range of sperm motile concentrations (Rappa et al. 2016; Nosrati et al. 2017).
In this study, we aimed to test a novel microfluidic channel-based biomimetic sperm selection device against the gold-standard, DGC, to isolate motile sperm from a range of semen samples. Our radial microfluidic device selects sperm based on their preference to follow boundaries in a static fluid environment mimicking the geometries of the female reproductive tract (see Figure 1). This device consists of a radial array of hundreds of media-filled channels, whereby semen interfaces with the entrances to these channels, selecting sperm by their ability traverse corners, boundaries and troughs, mimicking the endometrium and epithelium of the oviduct, towards a central outlet port. We hypothesized, based on our previous insights into motile sperm behavior in confined geometries and channels (Nosrati et al. 2014; Eamer et al. 2016; Vasilescu et al. 2023), that sperm boundary-following tendencies will lead to the isolation of a high motility, low-DFI sperm population.
Material and methods
Device Fabrication
The microfluidic device contained a semen reservoir designed to hold 1 mL of raw semen. The semen reservoir was connected to a sperm trapping and collection area via a radial array of microchannels (Figure 1 and Supplementary Video 1). Microfluidic devices were fabricated via a molding process using 3D printed molds adapted from Shrestha et al. (Shrestha et al. 2019). The molds were fabricated using a digital light processing (DLP) 3D printer, (MiiCraft Ultra 50, MiiCraft, Taiwan) and a photopolymer resin (BV-007, Creative CADWorks, Canada). The microfluidic device design was created in SolidWorks (2019) (SolidWorks Corp., USA) and sliced at 25 µm. The device was made from two separate molds comprising the top and bottom layers of the device. After printing, each mold was washed with isopropanol and dried with an air gun to remove any excess liquid resin from the microchannels. Washing with isopropanol was repeated three times and the molds then cured in a UV curing box for 2 minutes prior to being placed in a 70% ethanol bath for 2 hours. The molds were subsequently dried with an air gun and oxygen plasma treated (Basic Plasma cleaner PDC-002, Harrick Plasma) for 2 minutes, followed by salinization using trichloro (1H, 1H, 2H-perfluoro-octyl) silane (Sigma-Aldrich, Australia) in a desiccator under vacuum for 1.5 hours. The molds were cast with polydimethylsiloxane (PDMS), (Sylgard 184, Dow Corning, USA), a non-toxic polymer (Colucci et al. 2018), prepared using a mixture of base and curing agents in a ratio of 1:10. The mixture was degassed to remove all bubbles before casting onto the molds and cured hot air oven (75°C) for 2 hours. After curing, the PDMS layers were gently peeled off from the molds, holes punched for the semen inlet, overflow reservoir, and sperm collection outlet, and bonded through oxygen plasma treatment.
Semen Collection and Patient Demographics
Human semen samples were obtained through ejaculation after 2-4 days of sexual abstinence as recommended by the World Health Organization (WHO 2021). Raw semen samples were kept on a shaker at room temperature for 15-20 minutes to allow for full liquefaction. The proof-of-concept study was approved by the Monash University Human Research Ethics Committee (ID 26713) and the diagnostic andrology study was approved by the Melbourne IVF Human Research Ethics Committee (88-22-MIVF, approved May 2022). Samples were obtained from donors for the proof-of-concept study and from patients presenting for semen 6 analysis in the diagnostic andrology study. Specific age information is not available for the proof-of-concept study is not available, but these donors were students between the ages of 18 and 24, and patients in the diagnostic andrology study were between the ages of 26 and 54 (mean 36.3 years) (see Table 1). There are differences in the two populations, specifically in the average semen volume being higher in the diagnostic andrology group (P < 0.05). All participants provided informed consent to be included as completely de-identified participants in this study.
Exclusion criteria for both groups were azoospermic, severe oligozoospermic (<1 million/mL sperm concentration) or absolute asthenozoospermic patients (0% motile sperm). Participants providing samples of less than 1 mL volume and in the diagnostic andrology patients, those designated for cryopreservation. Finally, patients admitting to active infection or samples with signs of active infection or inflammation (presence of many round cells) were also excluded.
Experimental Procedure
61 patient and donor samples were processed across two separate studies. Both studies followed the same approach whereby all samples were split into three groups, raw semen, DGC and microfluidic device and processed in parallel. In cases where semen volume was less than 2.1 mL, the unprocessed sample was diluted to 2.1 mL in the GMOPS Plus (Vitrolife, Gothenburg, Sweden) to enable parallelised processing (9 out of 61 samples), as 1 mL was required for DGC, and 1 mL for the microfluidic device and 100 L of raw semen for as the control.
To perform sperm selection with the microfluidic device (see Figure 1Ai-iv), the device was pre-filled with room temperature Sydney IVF Gamete Buffer (Cook Medical, Bloomington, USA) for the proof-of-concept study or G-MOPS Plus media (Vitrolife, Gothenburg, Sweden) for the diagnostic andrology study by injecting 1.5 mL through the central outlet using a filled 3 mL plastic syringe (Becton, Dickinson and Company (BD), Franklin Lakes, USA). These two media are both routine sperm processing buffers at each site. A strip of AS110 acrylic medical grade adhesive tape (AR Care Ltd., Norfolk, United Kingdom) was then placed over the central outlet to create an airtight seal for channel stability preventing undesired flow of fluids. The device was then left for 5-10 minutes on a 37°C warm plate to 7 equilibrate. 1 mL of liquified semen was then injected into the device using a 1 mL BD plastic syringe and the device was left undisturbed at 37°C (on a heated stage) for 15 minutes. After incubation, the tape was removed and 200 µL of sperm suspended in media collected from the central outlet using a 200 µL pipette. The migration of sperm through various stages of the device can be seen in Supplementary Video 1.
In the proof-of-concept study DGC was performed using Sydney IVF 80/40 gradients (Cook Medical, Bloomington, USA). First, 1 mL of 40% gradient was placed in a conical 15 mL tube and under-laid with 80% gradient. Then, 1 mL of semen was carefully layered on top of the 40% density gradient layer using a 1 mL pipette. The solution was centrifuged at 500xg for 10 minutes and the pellet directly aspirated from the bottom of the tube by collecting 200 µL of fluid. The pellet was resuspended in 3 mL of Sydney IVF Gamete Buffer, centrifuged for 5 minutes at 500xg, and the subsequent pellet aspirated from the bottom by collecting 200 µL sperm in Sydney IVF Gamete Buffer and transferred to a final tube for analysis.
In the diagnostic andrology study DGC was performed using PureSperm 80/40 gradient (Nidacon, Gothenburg, Sweden). With aseptic techniques, 1 mL of 40% PureSperm density gradient was pipetted into a 15 mL conical tube. Using a new pipette, 1 mL of PureSperm 80% density gradient was carefully underlaid to avoid mixing the two layers. After carefully layering 1 mL of semen sample onto the gradient, without disrupting the density gradient, the gradient tube was centrifuged at 500xg for 10 minutes. After centrifugation, the gradient tube was carefully removed from the centrifuge to avoid mixing the layers. The pellet was removed using a clean Pasteur pipette and transferred to a clean 15 mL blue capped Falcon conical tube containing 8 mL of G-MOPS Plus media and centrifuged at 500xg for 5 minutes. Lastly, the pellet was transferred to the final tube with 200 µL G-MOPS Plus media. Therefore, at both sites the same media were used for both the microfluidic device and the wash centrifugation step of the DGC processing.
Sperm Chromatin Dispersion Assay and Motility Analysis
Assessment of DNA fragmentation index (DFI) was performed by a modified sperm chromatin dispersion (SCD) test, using the HT-HSG2 kit (Halotech DNA® , Madrid, Spain) as previously reported (Vasilescu et al. 2021). Sperm DNA fragmentation (SDF) was obtained for the initial raw semen sample and for sperm selected using the microfluidic device and DGC. Briefly, 80 μL of sperm suspension was added to a 2 mL Eppendorf tube (Eppendorf, Germany) and mixed with pre-warmed agarose, followed by 10 μL of the semen-agarose mixture being pipetted onto pre-coated slides and covered with a coverslip. The slides were placed on a cold plate at 4°C for 5 minutes to allow the agarose to set. The coverslips were then gently slid off the slides, and the slides immediately immersed horizontally in an acid solution and incubated for 7 minutes with a new cover slip placed on top. Slides were then gently tilted vertically to allow the acid solution to run off the slides. Slides were then horizontally immersed in the lysing solution for 20 minutes and washed with distilled water for 5 minutes, and then dehydrated in increasing concentrations of ethanol (70%, and 100%) for 2 minutes each, air-dried, and stored at room temperature in the dark. Sperm were categorized into one of five groups during counting following SCD. These were (i) Sperm with a halo width equal to or larger than the minor diameter of the core. (ii) Sperm with small halos, similar to or smaller than 1/3 of the minor diameter of the core. (iii) Sperm with medium halos, between small and large halos from groups (i) or (ii). (iv) Sperm with no halo. (v) Sperm with a degraded halo. Sperm with a small, degraded, or absent halo contained fragmented DNA (groups iv and v). DFI was recorded as the percentage of sperm cells with fragmented DNA and 300 sperm were counted per sample and each sample counted twice and considered an accurate count if the difference in DFI counts were within 5% of each other. 7 samples were removed from DFI results due to inadequate staining for reliable count.
Motility and concentration for each group was assessed using WHO 6 th Edition 2021 guidelines (WHO 2021), Sperm were classified as either progressively motile, nonprogressive, or immotile. The method for assessing sperm concentration and motility was performed consistently at both sites to ensure accurate comparisons and minimize confounding variables. For assessing sperm concentration, a 1:10 dilution of raw semen with gamete buffer (Sydney IVF Gamete Buffer for the proof-of-concept study and G-MOPS Plus for the diagnostic andrology study) was required for 10 of the 50 samples. Concentration was assessed under a phase contrast microscope using hemocytometer at 200X, and a duplicate 9 count was performed between two scientists, with counts repeated if the difference between each count exceeded 5%. For motility assessment, samples were assessed under similar conditions after liquefaction of semen within one hour of collection and immediately after processing with DGC and the microfluidic device. Sperm were classified as progressive, nonprogressive and immotile. A minimum of 200 sperm were assessed by two scientists with assessment repeated if the difference between each count exceeded 5%.
Statistical Analysis
All statistical analyses were performed using GraphPad Prism 6.0 (GraphPad Software, La Jolla, USA). Normal distribution was assessed using the Shapiro-Wilk Test. The statistical significance of the differences between the values was assessed using Friedmans test for nonparametric data to account for repeated measures from the same patient. Data is presented as median and interquartile range (IQR) and mean ± standard error of the mean (SEM) in Table 2. P < 0.05 was considered statistically significant.
Results
The radial microfluidic device selects sperm based on their preference to follow boundaries in a confined space within a static fluid environment, as shown in Figure 1B-ii and iii. Table 2 shows the sperm quality metric values for DFI, motility, and concentration for the three groups processed in parallel (raw semen, DGC, and microfluidic device) for both the proofof-concept study and the diagnostic andrology study
Proof-of-concept study
The proof-of-concept study showed the microfluidic device-selected sperm had significantly lower median DFI when compared to the DGC group (0.7% vs. 4.1% respectively, P < 0.01) (Figure 2A). The device also showed consistently lower DFI than DGC in all 21 samples, as the distribution of DFI values compared between split samples shows in Figure 2B. The microfluidic device yielded a 92.2% decrease in average DFI (calculated as the percentage reduction in DFI from the raw sample), outperforming DGC which reduced DFI by 57.4% on average. A significantly higher percentage of the microfluidic device-selected sperm were progressively motile than the DGC group (92.8% vs. 77.7% respectively, P < 0.01), showing 10 improvement over DGC in 20 of the 21 samples (Table 2, Figure 2C). This represents a twofold average increase in progressive motility in sperm selected by the microfluidic device compared to DGC (61.1% for microfluidic device and 30.5% for DGC). Microfluidic selection significantly reduced sperm concentration when compared to DGC selection (2.9 x 106 sperm/mL vs. 61.0 x 106 sperm/mL respectively, P < 0.0001) (Figure 2D). The concentration difference between raw semen and DGC was not significant. Each selection method resulted in a 200 µL suspension of sperm in gamete buffer. A before and after microfluidic device processing can be seen in Figure 2E and F respectively
Diagnostic andrology study
The diagnostic andrology study repeated trends observed in the proof-of-concept study in a population of patients presenting for infertility. Of the 33 samples processed for DFI, the microfluidic device-selected sperm had significantly lower median DFI when compared to the DGC group (1.0% vs. 3.9% respectively, P < 0.0001) and a reduction was seen in 30 of the 33 samples assessed (Table 2, Figure 3A). Although both the microfluidic device and DGC significantly reduce DFI when compared to the raw sample (P < 0.0001 and P < 0.01 respectively), the microfluidic device yielded an 82.9% average improvement (calculated as the percentage reduction in DFI from the raw sample), significantly outperforming DGC (P < 0.01) which reduced DFI by an average of 44.4%. Furthermore, irrespective of the DFI of a raw sample, microfluidic selected samples were reduced to less than 10% consistently; in comparison, DGC selection led to 10 of the 33 samples with higher than 10% DFI (Figure 3B). Unlike the proof-of-concept study, there was no significant difference in progressive motility of the sperm selected by the microfluidic device versus DGC methods and both microfluidic device and DGC significantly improved progressive motility when compared to the raw semen (Figure 3C, P < 0.0001 and P < 0.0001 respectively). Furthermore, sperm concentration reflected the similar results as the proof-of-concept study with microfluidic device selection yielding a lower median sperm concentration when compared to DGC selection (2.0 x 106 sperm/mL vs. 20.0 x 106 sperm/mL respectively, P < 0.0001) (Table 2, Figure 3D).
Site comparison
Results of each site were compared (Supplementary Figures 1A-C) and both the progressive motility (P < 0.0001) and concentration (P = 0.0015) of sperm selected by DGC was 11 significantly lower in the proof-of-concept study when compared to the diagnostic andrology study. There were no other significant differences observed between sites.
Discussion
In this study we demonstrate that passive biomimetic microfluidic channel-based processing of semen, from healthy donors as well as patients attending an IVF clinic for diagnostic andrology, enables the selection of a high proportion of progressively motile sperm with significantly lower DFI when compared to conventional DGC. A biomimetic mode of sperm selection offers consistent results and can be performed with minimal training, with the operation of the microfluidic device requiring only a syringe, a pipette and a heated stage or incubator to operate the device (Figure 1A and B). During the incubation time, only motile sperm make their way from the semen reservoir down the microchannels via boundary following behaviour towards the collection chamber, and in doing so, were resuspended in the gamete buffer (Figure 1A-iii). A sharp drop in height and gradually reducing channel height towards the centre of the device effectively limited the chance for sperm to exit the 200 µL collection zone. Passive sperm selection may also minimize iatrogenic DNA damage by avoiding any centrifugal forces and selects sperm based on previously reported boundaryfollowing behaviour which correlates with reduced DNA fragmentation (Denissenko et al. 2012; Eamer et al. 2016).
Our research group have tested a similar device against swim-up sperm selection on a smaller cohort of donors, which harnessed MACS® ART Annexin V beads (Miltenyi Biotec, Australia) and opposing neodymium magnetic plates (AMF Magnetics, Australia) to negatively select apoptotic sperm (Vasilescu et al. 2023). This previous device was more complex in operation, required multiple reagents, was fabricated from a different material (3D-printed photopolymer resin) and designed with different internal geometry. The current device uses a simpler, more accessible approach aimed at routine use. To test this microfluidic device, donor samples were used to compare DGC against the microfluidic method of sperm selection, which allowed selection of sperm with higher DNA integrity and progressively motile sperm from this cohort. While conventional semen processing with DGC did provide an average improvement in motility and DFI compared to unprocessed semen, it did so with a higher level of variability in sperm quality. Specifically, 3 of the 21 samples processed via DGC showed an increase in DFI and many samples only provided an 12 incremental reduction (less than 10%) (Supplementary Figure 2). Conversely, samples processed with the microfluidic device showed a significant improvement in all samples irrespective of starting value and motility. The average DNA fragmentation of sperm from the microfluidic device was less than 1%, demonstrating that this method of sperm selection, when applied to motile sperm populations, is effective regardless of the starting value for %DFI. Similarly, the motility of sperm recovered from the microfluidic device was consistently improved when compared to DGC (Figure 2C). These results were also consistent with previous studies indicating a high level of variance in recovered sperm motility from DGC (Malvezzi et al. 2014; Muratori et al. 2016).
When performing a study on 40 consenting diagnostic andrology patients, similar trends were observed. Despite being a more clinically diverse cohort, significant improvements in DFI were observed in 30 of the 33 samples assessed when isolating sperm using the microfluidic device as compared to DGC (two samples had identical DFI for both groups). This consistency highlights the usability and standardization achievable with a biomimetic device. Additionally, although overall improvements in DFI were observed using DGC (44.4% average), 3 samples showed an increase in DFI, possibly due to the iatrogenic damage caused by centrifugation on particularly susceptible samples but this would require further investigation (Supplementary Figure 3). This was not observed in the biomimetic device which showed an average DFI improvement of 82.9%, with only 1 sample showing less than a 60% reduction in DFI. Another noteworthy observation made in both studies was, although DFI was reduced in most samples for DGC selection, the average reductions in DFI of 57.4% and 44.4% for the proof-of-concept study and diagnostic andrology study respectively, are inefficient when compared to the 92.2% and 82.9% improvements observed in the biomimetic device selected groups. Compared to DGC, the microfluidic device increases the chance of selecting a high DNA integrity sperm, and this, creates a population of sperm for fertilization which has lower DNA damage and may provide clinical benefit within IVF workflows by reducing incidence of miscarriage and failed implantation as suggested in literature (Duran et al. 2002; Robinson et al. 2012; Sedó et al. 2017; Borges Jr et al. 2019).
The microfluidic device performed consistently between sites for all three key parameters measured (Supplementary Figures 1A-C). Although there are observable differences between DGC groups for progressive motility and concentration, these differences can be attributed to multiple factors including operator experience between research scientists at the university 13 research laboratory for the proof-of-concept study versus clinical embryologists in the diagnostic andrology study. Although there are differences in the density gradient used between the two sites, both gradients were a 40% and 80% gradient solution combination and are silane-coated silica-based.
The average %DFI after DGC varies within literature and depends largely on sample populations, with some studies indicating an increase in total DNA fragmentation (Muratori et al. 2019) and others suggesting an average improvement in DFI with a sub-population of samples experiencing an increase in DFI or no improvement in DFI, which is consistent with the results of this study (Stevanato et al. 2008; Rappa et al. 2016). DNA fragmentation in sperm is commonly attributed to oxidative stress, plausibly induced by repetitive centrifugation used in conventional sperm selection methods (Henkel et al. 2005; Nabi et al. 2014; Hernández-Silva et al. 2021). A high DNA fragmentation is associated with pregnancy loss in conventional IVF and ICSI, as well as lower implantation rates and a reduction in average embryo quality (Larson-Cook et al. 2003; Robinson et al. 2012; Coughlan et al. 2015; Benagiano et al. 2017). What is perhaps more concerning is that sperm DNA fragmentation has no obvious effect on fertilization but becomes apparent during blastocyst development by reducing the generation of good quality blastocysts and ability to achieve successful implantation (Seli et al. 2004; Newman et al. 2022). As a result, the risk of using compromised sperm remains present in clinical practice and highlights the need for the selection of sperm with high DNA integrity. Importantly, this is of relevance when considering that advanced reproductive age has an increased negative effect on sperm DNA damage (Horta et al. 2020). Furthermore, male ageing has been linked to a significant increase in miscarriages, and a decrease in live birth, with a larger impact in women of advanced reproductive age (Horta et al. 2019).
A clear limitation of the current device output, and arguably microfluidic motility-based sperm selection in general, is the smaller quantity of sperm isolated from the microfluidic device when compared to DGC. In conventional IVF, typically 50,000 sperm are required per oocyte and an average of 10-12 oocytes are harvested per stimulated cycle (Oseguera-López et al. 2019). Although it has been shown that high fertilization and cleavage rates with numbers as low as 2000-4000 sperm per oocyte is possible (Fiorentino et al. 1994). The average number of sperm from the microfluidic device was approximately 720,000, which may be too few for many conventional IVF cases if clinics were to adhere to a ~50,000 14 motile sperm per oocyte requirement (Li et al. 2018). Logically speaking, sperm selected through passive biomimetic selection such as the device in this study, may have higher fertilisation efficiency than those selected with active measure such as centrifugation, therefore fewer sperm are required for conventional IVF, similar to that of in vivo fertilisation whereby only approximately 200 sperm fertilise the oocyte (Chaffey 2003). Nevertheless, in future prototypes of this biomimetic device, improvement in the yield of motile sperm for higher responding women with many oocytes collected will improve the potential for clinical adoption.
Semen processing via DGC requires several manual interventions during sample handling, each with the potential for human error. Passive devices like the one used in this study as well as ZyMōt ® (ZyMōt Fertility, New York City, USA) and Lenshooke CA0® (Hamilton Thorne, Beverly, USA) limit human interaction with semen to sample injection and sperm collection, usually without centrifugation (Shirota et al. 2016). The microfluidic device used here, with a simple 3-step operation, will reduce the clinical workload while offering improved DFI reduction after processing. While many studies have investigated the impacts of commercialized microfluidic devices which have been systematically reviewed (Ferreira Aderaldo et al. 2023), the present biomimetic device takes a different approach to sperm selection by leveraging the boundary following behavior of sperm to perform selection, and requiring sperm to travel several millimeters to a collection zone. DNA fragmentation reductions and the simplicity of this device are comparable to existing commercial devices by ZyMōt® and LensHooke® (Parrella et al. 2019; Hsu et al. 2023), which both exploit sperm motility via membrane filtration. In a recent study comparing DGC, ZyMōt® , and the LensHooke CA0® , Hsu et al demonstrated progressively motile sperm counts of 80.6%, 85.6%, and 90.8%, respectively and DFI measurements of 11.8%, 3.7%, and 2.4% in normospermic samples (Hsu et al. 2023). Further comparative studies are now required to determine whether using a biomimetic that leverages boundary following behavior in sperm will lead to improved outcomes.
There are several limitations to this study which can be addressed in larger follow-up studies. Firstly, the limited access to samples with high DFI (>25%), prevented the robust testing approach on extreme cases and perhaps those which would benefit the most from a reduction in DFI. Secondly, to prove the clinical usefulness of this approach, clinical outcomes are required when assessing the device on a range of patients whereby the effect of each sperm 15 selection method on fertilisation and embryo development is thoroughly evaluated. Ideally, a randomized controlled trial or sister oocyte study (whereby half the oocytes are inseminated with sperm isolated using conventional methods and half the oocytes are inseminated with sperm isolated with the microfluidic device) will better display the clinical utility of this microfluidic device in IVF workflows. The prototype in its current format, does show utility for ICSI cases whereby high-quality sperm in lower numbers is sufficient, and this format suits a side-by-side study for an ICSI cohort but may not suit an IVF insemination side-byside comparison with DGC. Thirdly, the method of DNA fragmentation assessment, SCD, only identifies single-stranded DNA breaks, and has limitations in the subjective and nature of the assessment, and future studies and validation will be performed using SCSA for a more robust assessment of DFI by detecting double-stranded DNA breaks. SCD also is susceptible to human error and sperm concentration restraints during the preparation and staining of samples, shown in 7 of the 40 patients unsuitably stained for SCD assessment. Finally, the current biomimetic prototype does limit output of sperm by only processing 1 mL of semen compared to the entire ejaculate being processed by conventional methods. The purpose of this was to enable side-by-side testing against DGC, however further studies are currently evaluating a larger volume platform capable of processing an entire semen sample to maximize sperm yield for use in IVF and IUI.
Here we provide a novel, highly selective biomimetic method of sperm selection in a simpleto-use, single-use chip format, for isolating highly motile sperm with minimal DNA fragmentation, without the need for centrifugation or other active mechanisms. Considering the limitations of this study, this proof-of-concept testing shows that highly selective, lower output sperm isolation such as channel-based microfluidic selection in its current form, may prove a practical alternative for ICSI cycles if higher motile concentrations for larger oocyte numbers are preferred for conventional IVF. The novel selectiveness of mimicking the female reproductive system provides a high-quality population of sperm for use in treatments. Clinical studies have now been initiated to validate the proposed benefits of this selection mechanism.
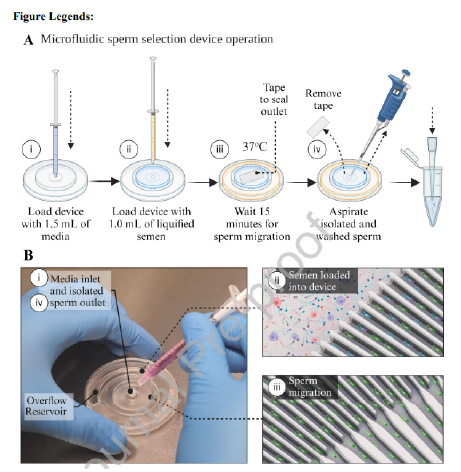

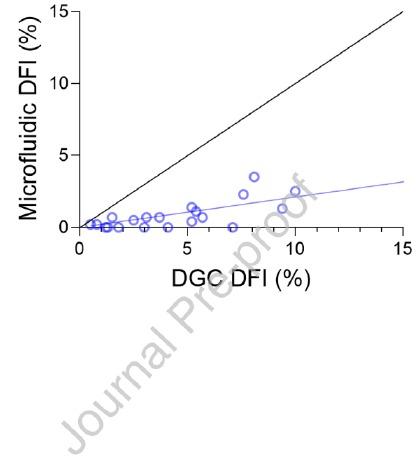


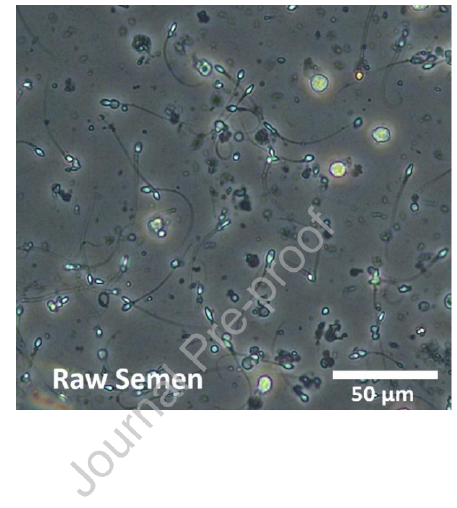
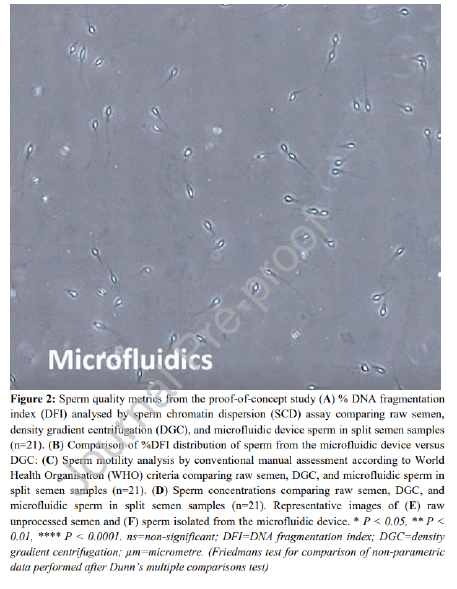
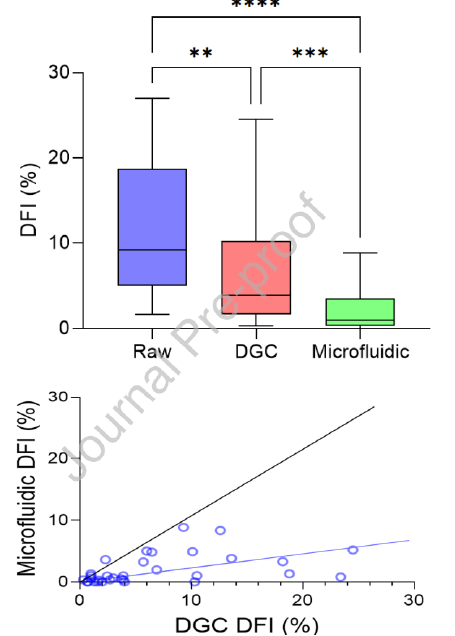
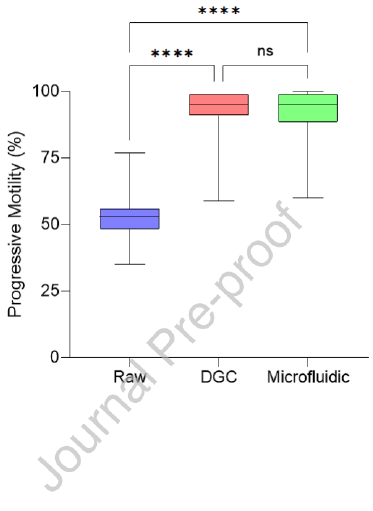
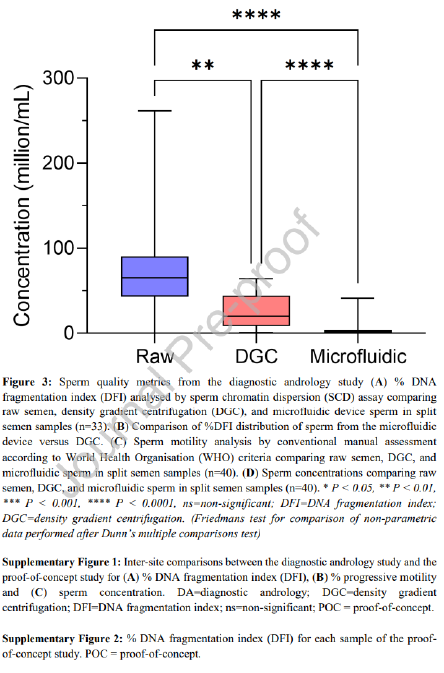
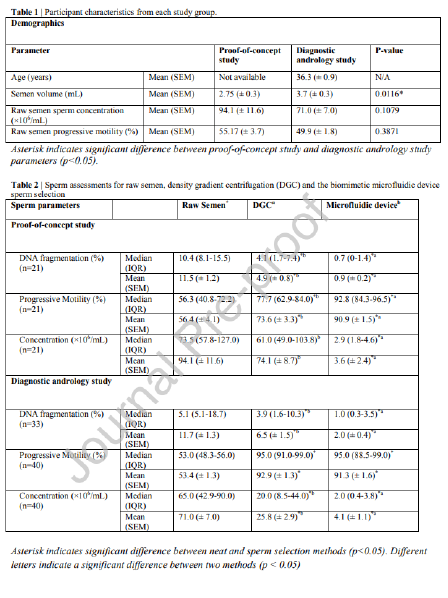
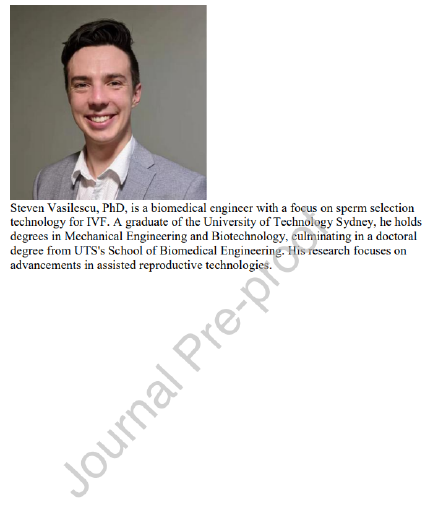